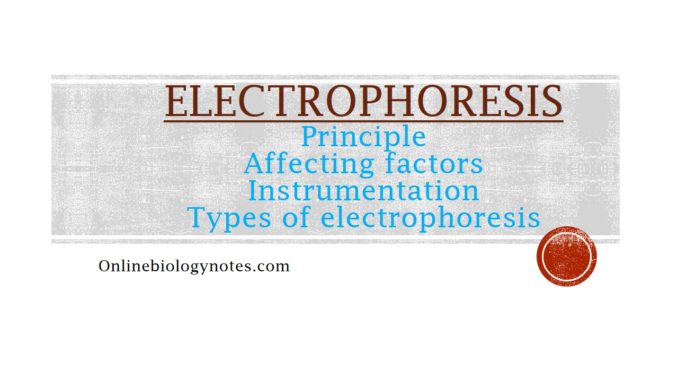
What is electrophoresis?
- The term electrophoresis describes the migration of a charged particles under the influence of an electric field.
- Various essential biological molecules, such as amino acids, peptides, proteins, nucleic acids, nucleotides, have ionizable group, which at given pH exist in a solution as electrically charged species either as cation (+ve) and anion (-ve) are separated by electrophoresis
- Under the influence of electric field these charged particles will migrates either to cathode or anode depending on the nature of their net charge
Principle of electrophoresis:
- When a potential difference is applied, the molecules with different overall charge will begin to separate owing to their different electrophoretic mobility. Even the molecules with similar charge will begins to separate if they have different molecular sizes, since they will experience different frictional forces. Therefore, some form of electrophoresis rely almost totally on the different charges on the molecules for separation while some other form exploits difference in size (molecular size) of molecules.
- Electrophoresis is regarded as incomplete form of electrolysis because the electric field is removed before the molecules in the samples reaches the electrode but the molecules will have been already separated according to their electrophoretic mobilities.
- The separated samples are then located by staining with an appropriate dye or by autoradiography, if the sample is radiolabeled.
Factor affecting electrophoresis:
i. Nature of charge:
- Under the influence of an electric field these charged particles will migrate either to cathode or anode depending on the nature of their net charge.
ii. Voltage:
- When a potential difference (voltage) is applied across the electrodes, it generates a potential gradient (E), which is the applied voltage (v) divided by the distance “d” between the two electrodes i.e. p.d. (E) = V/d.
- When this potential gradient ‘E’ is applied, the force as the molecule bearing a charge of ‘q’ coulombs is ‘Eq’ Newtons.
- It is this force that drives the molecule towards the electrodes.
iii. Frictional force:
- There is also a frictional force that retards the movement of this charged molecule.
- This frictional force is the measure of the hydrodynamic size of the molecule, the shape of the molecule, the pore size of the medium in which the electrophoresis is taking place and the viscosity of the buffer.
- The velocity ‘v’ of the charged molecule is an electric field is therefore given by the equation. U=Eq/f, where ‘f’= frictional coefficient
iv. Electrophoretic mobility:
- More commonly a term electrophoretic mobility ( ) of an ion is used, which is the ratio of the velocity of the ion and the field strength. i.e. =U/E.
- When a p.d. is applied, the molecule with different overall charges will begin to separate owing to their different electrophoretic mobility.
- Even the molecule with similar charges will begin to separate if they have different molecular sizes, since they will experience different frictional forces.
V. current:
- Ohm’s law: V/I=R
- It therefore appears that it is possible to accelerate an electrophoretic separation by increasing the applied voltage, which ultimately results in corresponding increase in the current flowing.
- The distance migrated by the ions will be proportional to both current and time.
vi. Heat:
- One of the major problems for most forms of electrophoresis, that is the generation of heat.
- During electrophoresis, the power (W) generated in one supporting medium is given by W= I2R
- Most of the power generated is dissipated as heat.
- The following effects are seen on heating of the electrophoretic medium has:
- An increased rate of diffusion of sample and buffer ions which leads to the broadening of the separated samples.
- Formation of convention currents, which leads to mixing of separated samples.
- Thermal instability of samples that are sensitive to heat.
- A decrease of buffer viscosity and hence reduction in the resistance of the medium.
- If a constant voltage is applied, the current increases during electrophoresis owing to the decrease in resistance and this rise in current increases the heat output still further.
- For these reasons, often a stabilized power supply is used, which provides constant power and thus eliminates fluctuations in heating.
- Constant heat generation is however a problem. For which the electrophoresis is run at very low power (low current) to overcome any heating problems, but this can lead to poor separation as a result of the increased amount of diffusion due to long separation time.
- Compromise condition have to be found out with reasonable power settings, to give acceptable separation time and an appropriate cooling system, to remove liberated heat. While such system works fairly well, the effect of heating are not always totally eliminated.
Vii. Elecrtroendosmosis:
- The phenomenon of electroendosmosis (aka- electro-osmotic flow) is a final factor that can affect electrophoretic separation.
- This phenomenon is due to the presence of charged groups on the surface of the support medium.
- For instance, paper has some carboxyl group present, agarose contains sulfate groups depending on the purity grade and the surface of glass walls used in capillary electrophoresis contains silanol (Si-OH) groups.
- These groups, at appropriate pH, will ionize, generating charged sites.
- It is these charges that generate electroendosmosis.
- In case of capillary electrophoresis, the ionized sianol groups creates an electrical double layer, or a region of charge separation, at the capillary wall/electrolytic interface.
- When voltage is applied cations in the electrolyte near the capillary walls migrate towards the cathode, pulling electrolyte solution with them.
- This creates a net electroosmotic flow towards cathode.
Types of support media used in electrophoresis:
- The earliest supports used were filter paper or cellulose acetate strips, wetted in electrophoresis buffer. Nowadays these media are not is use.
- Nowadays either an agarose gel or polyacrylamide gels are used.
- Agarose gel:
- Agarose- a linear polysaccharide (M.W. 12000 Da) made up of the basic repeat unit of agarobiose (which comprises alternating units of galactose and 3,6-anhydrogalactose.
- It is one of the components of agar, that is a mixture of polysaccharides from seaweeds.
- It is used at a concentration between 1% and 3%.
- Agarose gel is formed by suspending dry agarose in aqueous buffer and then boiling the mixture till it becomes clear solution, which is then poured and allowed to cool at room temperature to form rigid gel.
- The gelling properties is attributed to inter and intramolecular H-bonding within and between long agarose chains.
- The pore size of the gel is controlled by the initial concentration of agarose, large pore size corresponds to low concentration and vice versa.
- Although free from charges, substitution of the alternating sugar residues with carboxyl, methoxyl, pyruvate, and sulfate groups occur to varying degrees which can result in electroendosmosis during electrophoresis.
- Agarose is therefore sold in different purity grades, based on the sulfate concentration- the lower the sulfate concentration, the higher the purity.
- These gels are used for the electrophoresis of both proteins and nucleic acids.
- For proteins, the pore size of a 1% agarose gel is large relative to the sizes of proteins.
- Therefore, used in techniques such as immune-electrophoresis or flat-bed isoelectric focusing, where proteins are required to move unhindered in the gel matrix according to their native charge.
- Such large pure gels are also used to separate much larger molecules such as RNA and DNA, because the pore sizes are still large enough for RNA and DNA molecule to pass through the gel.
- An advantage of using agarose in the availability of low melting point agarose (62-65oC).
- This gel can be reliquefied by heating to 65oC and thus, for example DNA samples separated can be cut out of the gel, returned to solution and recovered.
- Polyacrylamide gel:
- Cross-linked polysaccharide gel are formed from the polymerization of acrylamide monomer in the presence of small amount of N,N’-methylene bis acrylamide (aka- bis-acrylamide).
- Bis-acryl amide is basically two acrylamide molecules linked by a methylene group, and is used as a cross-linking agent.
- Acrylamide monomers is polymerized in head to tail fashion into long chain, thus introducing a second site for chain extension.
- Proceeding in this way, a cross-linked matrix of fairly well-defined structure is formed.
- The polymerization of acrylamide is an example of free radical catalysis and is initiated by the addition of ammonium persulfate and the base N, N, N’, N’- tetra-methylene diamine (TEMED).
- TEMED catalyses decomposition of the persulphate ion to give free radical.
- S2O82- + e– à SO42- + SO-•4
- R• + M à RM•
- RM•+ M à RMM•
- RMM•+M à RMMM• and so on…
- Photopolymerisation is an alternative method that can be used to polymerize acrylamide gels.
- The ammonium persulphate and TEMED are replaced by riboflavin and when the gel is poured, it is placed in front of a bright light for 2-3hrs.
- Photodecomposition of riboflavin generates a free radical that initiates polymerization.
- Acrylamide gels are defined in terms of the total percentage of acrylamide present, and the pore size in the gel can be varied by changing the concentration of both acrylamide and bis-acrylamide.
- The acrylamide gel can be made with a content between 3% and 30% acrylamide.
- Thus, the low percentage gels (e.g., 4%) have large pore size and are used for electrophoresis of protein- example flat bed isoelectric focusing, or stacking gel system of an SDS-PAGE.
- Low percentage acrylamide gels are also used to separate DNA.
- Gels between 10% and 20% acrylamide are used in techniques such as SDS-gel electrophoresis, where smaller pore size now introduces a sieving effect that contributes to the separation of proteins according to their size.
Instrumentation of electrophoresis:
- Equipment required for electrophoresis basically consists of two items, a power pack and an electrophoresis unit.
- Electrophoresis units are available for running either vertical or horizontal gel systems.
- Vertical slab gel units are commercially available and routinely used to separate proteins in acrylamide gels.
- The gel is formed between two glass plates, clamped together but held apart by plastic spacers.
- Gel dimensions are typically 12cmX 14cm, with a thickness of 0.5 to 1mm.
- A plastic comb is placed in the gel solution and is removed after polymerization to provide loading wells for samples.
- When the apparatus is assembled, the lower electrophoresis, tank buffer surrounds the gel plates and affords some cooling of the gel plates.
- The gel is cast on a glass or plastic plates and placed on a cooling plate (an insulated surface through which cooling water is passed to conduct away generated heat.)
- Connection between the gel and electrode buffer is made using a thick wad of wetted filter paper, however the agarose gels for DNA electrophoresis are run submerged in the buffer.
- The powerpack supplies a direct current between the electrodes in the electrophoresis unit.
- All electrophoresis is carried out in an appropriate buffer, which is essential to maintain a constant state of ionization of the molecules being separated.
- Any variation in pH would alter the overall charge and hence the mobilities (rate of migration in the applied field) of the molecules being separated.
Different types of electrophoresis:
1. Sodium dodecyl sulphate-polyacrylamide gel electrophoresis (SDS-PAGE):
- Most widely used method for analyzing protein mixture qualitatively, particularly useful for monitoring protein purification and because the method is based on the separation of protein on the basis of size, thus can also be used to determine the relative molecular mass of proteins.
- SDS (CH3-(CH2)10-CH2OSO3–Na+) is an anionic detergent.
- Samples to be run on SDS-PAGE are firstly boiled for 5 min in sample buffer containing beta-mercaptoethanol and SDS.
- Mercaptoethanol reduces the di-sulphide bridges present that holds the tertiary structure of protein, therefore by this treatment, the protein opens up into a rod-shaped structure with a series of -vely charged SDS molecules along the polypeptide chain.
- The original native charge on the molecule is therefore completely swamped by the -vely charged SDS molecules.
- The rod-like structure remains as any rotation that tries to fold up the protein chain would yield in repulsion between negatively charged on various parts of protein chain, returning the conformation to the rod shape.
- The sample buffer also includes an ionizable tracking dye, usually bromophenol blue, that permits the electrophoretic run to be examined, and sucrose and glycerol, which provides the sample solution density.
- This allows the sample to settle easily through the electrophoresis buffer to the bottom when injected into the loading well.
- The sample to be separated are not loaded directly into the main separating gel, rather it is loaded into a shorter (approx.1cm) stacking gel, which is poured on top of the separating gel.
- The purpose of the stacking gel is to concentrate the protein sample into sharp band before it enters the main separating gel, which is achieved by utilizing the difference in ionic strength and pH between the electrophoresis buffer and the stacking gel and involves a phenomenon k/a iso-tachophoresis.
- The stacking gel has a very large pore size (4% acrylamide), which allows the proteins to move freely and concentrate or stack, under the effect of the electric field.
- The band sharpening effect depends on the fact that -vely charged glycinate ions (electrophoresis buffer) have lower electrophoretic mobility than the protein SDS complexes, which in turn have lower mobility than the chloride ions (Cl–) of the loading buffer and the stacking gel.
- When the current is switched on, all the ionic species have to migrate at the same speed otherwise there would be a break in the electrical circuit.
- The glycinate ions can move at the same speed as Cl– only if they are in a region of higher field strength.
- Field strength is inversely proportional to conductivity, which is proportional to concentration.
- The result is that 3 species adjust their concentration so that [Cl–]> [protein-SDS] > [glycinate].
- There is only a small quantity of protein-SDS complexes so they concentrate in a very tight band between glycinate and Cl– boundaries.
- Once the glycinate reaches the separating gel it becomes more fully ionized in the higher pH environment and its mobility increases (pH-stacking gel 6.8 and separating gel 8.8).
- Thus, the interface between glycinate and Cl– leaves behind the protein-SDS complexes, which are left to electrophorese at their own rates.
- The -vely charged protein-SDS complexes now continue to move towards the anode, and because they have the same charge per unit length, they travel into the separating gel under the applied electric field with the same mobility.
- But, as they pass through the separating gel, the proteins separate as per the molecular sieving properties of the gel.
- Quite simply, the smaller the protein the more easily it can pass through the pores of the gel, whereas large proteins are successively retarded by frictional resistance die to the sieving effect of the gels.
- The bromophenol blue dye is totally unretarded as it is small molecule and therefore indicates the electrophoresis front.
- The current is turned off when the dye reaches the bottom of the gel, and the gel is eliminated from between the glass plates and shaken in an appropriate stain solution (usually Coomassie brilliant blue) for few hours and then washed in destain solution overnight.
- The destain solution eliminates the unbound background dye from the gel leaving stained proteins visible as blue bands on a clear background.
- A typical gel would take 1 to 1.5 hours to prepare and set, 3hours to run at 30mA, and have staining time of 2-3hrs with an overnight destain.
- Typical, separating gel used 15% polyacrylamide gel. This gives gel of certain pore size in which proteins of relative molecular mass (Mr) 10,000 move through the gel relatively unhindered.
- SDS-PAGE is often used after each step of purification protocol to assess the purity.
2. Iso-electric focusing gel (IEF):
- It is ideal for separation of amphoteric substance such as proteins because it is based on the separation of molecules according to their different iso-electric points.
- Has high resolution, being able to separate proteins that differ in their iso-electric points by as little as 0.01 of a pH unit.
- Most widely used system for IEF utilizes horizontal gels on glass plates or plastic sheets.
- Separation is achieved by applying a potential difference across the gel that contains a pH gradient, which is formed by the introduction of the ampholytes (complex mixtures of synthetic polyamino-polycarboxylic acids).
- Ampholytes are available in different pH ranges (pH 3 to 10) and a pH range is chosen such that the sample being separates will have their isoelctric point within this range.
- Commercially available ampholytes includes bio-lyte and pharmalyte.
- Traditionally, 1-2mm thick isoelectric focusing gel have been used, but nowadays, thin layer IEF gels of only 0.15mm thick, are used, prepared using a layer of electrical insulation tape as the spacers between the gel plates.
- Since, this method requires the proteins to move freely according to their charge under the electric field.
- To avoid any sieving effect within the gel, IEF is employed in low percentage gels.
- Commonly, 4% polyacrylamide gel are used, but agarose is used, for the study of high relative molecular mass proteins that may undergo some sieving even in a low percentage acrylamide gel.
- Thin layer IEF gel is prepared by mixing ampholytes, gel material and riboflavin and the mixture is then poured over a glass plate (25cmX10cm).
- The second glass plate is then placed on top of the first to form gel cassette and the gel polymerization is carried out by photopolymerization by placing the gel in front of a bright light.
- This takes 2-3hrs.
- After the gel is set, the glass plates are removed apart to reveal the gel stuck to one of the glass sheets.
- Electrode wicks (3mm) which are strips of wetted filter paper (anode-phosphoric acid and cathode-sodium hydroxide) are laid along the long length of each side of the gel and a potential difference is applied.
- Under the effect of this potential difference, the ampholytes forms a pH gradient between anode and a cathode.
- The power is then turned off and samples is applied by laying on the gel small squares of filter paper soaked in the sample.
- A voltage is again applied for about 30min to allow the sample to electrophorese off the paper into the gel, at which time the paper squares can be removed from the gel.
- Depending on which point on the pH gradient, the sample has been loaded, proteins that are initially at pH regions below their isoelectric point will be +vely charged and migrate towards cathode.
- As they proceed, the surrounding pH will increase steadily and the +ve charge on protein will decrease correspondingly until eventually the protein arrives at Zwitter ion form with no net charge, hence the further movement ceases.
- Similarly, the proteins at pH region above their iso-electric point will be -vely charged and will migrate towards the anode until they reach their iso-electric points and become stationary.
- To achieve rapid separation (2-3hrs) relatively high voltage (up to 2500V) are used.
- Following electrophoresis, the gel must be stained to detect the proteins, as this cannot be done directly, because the ampholytes will stain too, giving totally blue gel.
- So, for this purpose, the gel is therefore washed with fixing solution (10% v/v trichloroacetic acid), which precipitates the proteins and allows much smaller ampholytes to be washed out.
- The gel is then stained with Coomassie brilliant blue and then destained.
- The pI of a particular protein may be determined conveniently by running a mixture of proteins of known isoelectric point on the same gel.
- After staining, the distance of each band from the electrode is measured, and a graph of distance for each protein against its pI is plotted and by this calibration line, the pI of unknown protein can be determined.
3. Cellulose acetate electrophoresis
- One of the older methods, and has a number of applications particularly used in the clinical analysis of serum samples.
- Has the advantage over the paper in that it is a much more homogeneous medium, with uniform pore size and doesn’t absorb proteins like the paper.
- Far simpler to set up and run, single samples are normally run on cellulose acetate strips (2.5 X 12 cm), although multiple samples are frequently run on wider sheets.
- The cellulose acetate is first method in electrophoresis buffer (pH 8.6 for serum) and 1 to 2 l sample is loaded.
- The end of the strip makes contact with the electrophoresis buffer tanks via a filter paper wick and electrophoresis is conducted at 6-8 V/cm for about 3hrs.
- Following electrophoresis, the strip is stained for protein, destained, and the band visualized.
- A typical serum protein separation shows about 6 major bands in contrast to it, in disease states, this serum protein profile changes and a clinical can obtain information concerning the disease state of a patient from the altered pattern.
4. Pulse field gel electrophoresis (PFGE):
- PFGE is used for separation of DNA fragments up to 2X 103kb, it, therefore, allows the separation of whole chromosomes by electrophoresis.
- Method basically involves electrophoresis in agarose where two electric fields are applied alternately at different angles for defined time periods (60secs).
- The coiled molecules become stretched in the horizontal plane by the activation of the first electric field and start to move through the gel.
- The interruption of this field and application of the second field force the molecule to move in the new direction.
- Since there is a length-dependent relaxation behavior when a long chain molecule undergoes conformational changes in an electric field, the smaller the molecule, the quicker it re-aligns itself with the new field and is able to continue moving through the gel.
- Larger molecules take longer to re-align. In this way, with continual reversing of the field, smaller molecules draw ahead of larger molecules and separate according to size.
5. Agarose gel electrophoresis of DNA:
- Used for separation of DNA molecules, which are considerably larger than proteins and as most DNA fragments would be unable to enter a polyacrylamide gel, the larger pore size of an agarose gel is required.
- Since, the charger per unit length (due to PO4 group) in any given fragments of DNA is the same, all DNA samples should move towards the anode with the same mobility under an applied electrical field.
- Separation in agarose gel is achieved due to resistance to their movement caused by the gel matrix.
- The largest molecules will have most difficulty passing through the gel pores where as small molecules will be relatively unhindered.
- Consequently, the mobility of DNA molecules during gel electrophoresis will depend on size, the smallest molecule moving fastest.
- Gels containing 0.3% agarose will separate DNA molecules between 5 and 60kb size, where as 2% gels are used for samples of between 0.1 and 3Kb.
- Routinely use 0.8% gels, which are suitable for separating DNA molecules in the range of 0.5 to 10Kb.
- DNA gels are invariably run as horizontal, submarine, submerged gels, so named because such a gel is totally immersed in buffer.
- Agarose dissolved in gel buffer by boiling, is poured into a glass or plastic plates, surrounded by a wall of adhesive tape or a plastic frame to provide a gel about 3mm in depth.
- Loading wells are formed by placing a plastic well-forming template or comb in the poured gel solution, and the comb is removed after the gel is set.
- The gel is placed in electrophoresis tank, covered with buffer, and sample loaded by directly injecting the sample into the wells.
- Samples are prepared by dissolving them in a buffer solution that contains sucrose, glycerol and ficoll, which makes the solution sense and allows it to sink to the bottom of the well.
- A dye such as bromophenol blue is also included in the solvent, to monitor sample loading and marker of the electrophoresis front.
- No stacking gel is needed for the electrophoresis of DNA because the mobilities of DNA molecules are much greater in the well than in gel and therefore all molecules in the well pile up against the gel within a few minutes of current being turned on, forming a tight band at the start of the run.
- Once the system has been run, the DNA in the gel needs to be stained and visualized.
- The reagent most widely used is the fluorescent dye ethidium bromide.
- The gel is rinsed gently in a solution of ethidium bromide and is viewed under UV light (300nm), the DNA bands fluoresce orange-red (binds with/ intercalates between the stacked base pairs of DNA).
- As little as long of DNA can be visualized as a 1cm wide band, excessive viewing of DNA with UV light can result in damage of DNA with UV light can result in damage of DNA by nicking and base-pair dimerization.
6. Two-dimensional polyacrylamide gel electrophoresis:
- Combines the techniques of IEF, which separates proteins in a mixture according to charge (pI) with the size separation technique of SDS-PAGE.
- When combined to give two-dimensional PAGE, the most sophisticated analytical method for separating proteins available is obtained.
- The first dimension (IEF) is carried out in polyacrylamide gels in narrow tubes (Internal diameter 1-2mm) in presence of ampholytes, 8M urea and non-ionic detergent.
- The gel is then extruded from the tube by applying slight pressure to one end, incubated for 15mins in a buffer containing SDS, then placed along the stacking gel of an SDS -added gel and fixed in a place by pouring molten agarose, in electrophoresis buffer over the gel,
- Once the agarose has set, electrophoresis is commenced and the SDS bound proteins run into gel and are separated according to size.
- Is capable of routinely resolving between 1000 and 2000 proteins from a whole cell or tissue extract.