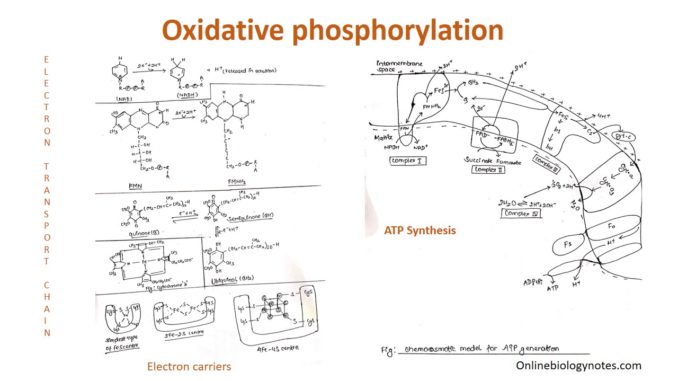
Oxidative phosphorylation:
- Reducing equivalent NADH, FADH2 generated during glycolysis and the link between glycolysis and Kreb’s cycle are used to synthesize ATP by a process called oxidative phosphorylation (OP).
- Oxidative phosphorylation involves two components-
- Electron transport chain
- ATP synthase.
- The flow of electrons from the reducing equivalence across the electron transport chain generates proton motive force (PMF).
- The energy stored in proton motive force is used to drive the synthesis of ATP.
- ATP synthase utilizes this proton motive force to drive the synthesis of ATP.
Electron transport chain:
- Electron transport chain consists of the series of electron carriers arranged asymmetrically in the membrane.
- The membrane may be either cytoplasmic membrane as in the case of bacteria or inner mitochondrial membrane as in case of eukaryotes.
- The electron carriers are sequentially arranged and get reduced as they accept electron from the previous carrier and oxidized as they pass electron to the succeeding carrier.
- The different electron carriers are:
- NADH dehydrogenase
- Flavoproteins (FMN and FAD)
- Ubiquinone
- Iron sulfur (Fe) center
- Cytochrome
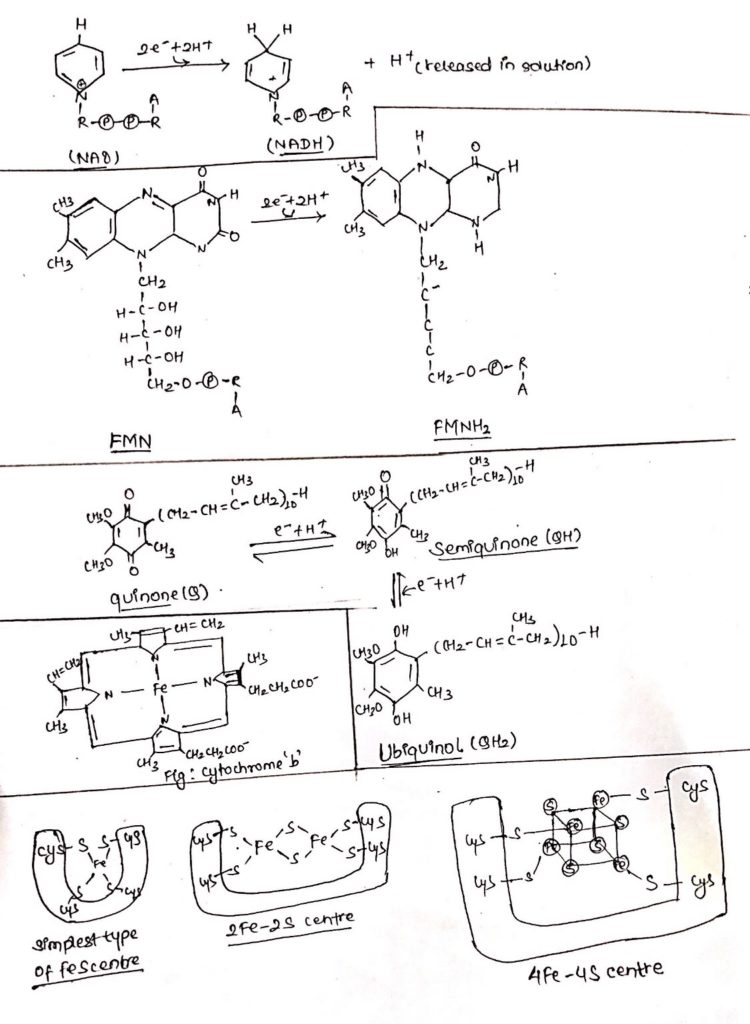
1. NADH dehydrogenase:
- Two types of NAD dependent dehydrogenase can feed electron transport chain.
- They are NADH and NADPH.
- NADPH is less common as it is involved in anabolic reactions (biosynthesis).
- NADH dehydrogenase removes two hydrogen atoms from the substrate and donates the hydride ion (H–) to NAD+ forming NADH and H+ is released in the solution.
- NAD+ accepts two e– and two protons from the substrate during catabolic reaction and transfers to the electron transport chain.
- NAD+ is then reduced to NADH+ H+.
- Reduced NADH+ H+ transfers its e– and proton to FMN which in turn is reduced to FMNH2.
- AH2+ NAD+ <——————–>A + NADH + H+
- (Reduced substrate) (oxidized substrate)
- NADH + H+ + FMN
<———–> FMNH2+ NAD+
2. Flavoproteins:
- Flavoproteins are derived from Vitamin B2 (Riboflavin).
- These are the protein containing FMN and FAD as the prosthetic group which may be covalently bound with the protein.
- They are capable of accepting electrons and protons but can only donate electrons.
- The protons are expelled outside the membrane.
- FMN accept electron and proton from NADH and get reduced to FMNH2 which in turn channel only e– through to ubiquinone.
- FAD is the component of succinate dehydrogenase complex.
- It accepts two electron and two protons from succinate and gets reduced to FADH2, in the process succinate is converted to fumarate.
- FADH2 channels its electron only to FeS center through ubiquinone.
- Succinate+ FAD ____________________> Fumarate + FADH2
3. Ubiquinone:
- Ubiquinone are omnipresent in nature.
- These are similar in structure and property with Vitamin K.
- In plants, these are found as plastoquinone and in bacteria, these are found as menaquinone.
- These are lipid soluble (hydrophobic) and can diffuse across the membrane and channel electrons between carriers.
- Ubiquinone can accept electrons as well as protons but transfer only electrons.
- They accept electron from complex 1 and 2.
- They can accept one e– and get converted into semiquinone or two e–s to from quinone.
4. FeS center:
- These are non-heme Fe (iron) containing proteins in which the Fe-atom is covalently bonded to Sulphur of cysteine present in the protein and to the free Sulphur atoms.
- Less commonly found FeS centers known as Reiske iron sulphur centers have iron bonded to Histidine residue of the proteins.
- There are different types of iron Sulphur center, simplest type consists of an iron atom, another type known as 2Fe-2S (Fe2S2) and the third one (most commonly found) is 4Fe-4S (Fe4-S4) and comprises the ferredoxin.
- FeS center consists of Fe-atoms which can interconnect between ferrous and ferric form as they accept and donate electrons respectively.
- They are capable of receiving and donating electrons only.
- They form the components of all four complexes.
5. Cytochromes:
- Cytochromes are the proteins with characteristic absorption of visible lights due to the presence of heme containing Fe as co-factor.
- There are three different types of cytochrome a, b and c.
- Cytochrome a and b are tightly but not covalently linked with their proteins whereas cytochrome c is covalently bonded with its protein through cysteine.
- Cytochrome ‘a’ has the maximum absorption spectra at 600nm.
- Cytochrome ‘b’ has maximum absorption spectra at 560nm and cytochrome ‘c’ has maximum absorption spectra at 550nm.
- Cytochromes are capable of accepting and transferring only one e– at a time during which the Fe– atoms interconvert between ferrous and ferric.
- Cytochrome- Fe2+ <————> Cytochrome- Fe3+ + e–
- Cytochromes are arranged in the order cytochrome ‘b’, cytochrome c1, cytochrome ‘c’ and cytochrome a/a3.
- a/a3 is also known as cytochrome oxidase.
Arrangement of five electron carriers in the form of four respiratory enzyme complex
- The five electrons carriers are arranged in the form of four complexes.
- Complex I: NADH Quinone oxidoreductase complex (NADH to Quinone)
Note: NADH——->FMN——> FeS—–> Q - Complex II: Succinate dehydrogenase complex (Succinate to Quinone)
Note: Succinate——> FAD—–> FeS—-> Q - Complex III: cytochrome bc1 (Ubiquinone to cytochrome c)
Note: UQ2——> cyt bc1—->cyt c - Complex IV: Cytochrome oxidase (cytc to O2)
Note: cyt c—-> cyt a—–> cyt a3—-> O2
- Complex I: NADH Quinone oxidoreductase complex (NADH to Quinone)
Complex I: NADH dehydrogenase complex
- This complex is also known as NADH dehydrogenase complex, consists of 42 different polypeptides, including FMN containing flavoprotein and at least six FeS centers.
- Complex I is ‘L’ shaped with its one arm in the membrane and another arm extending towards the matrix.
- During catabolic reaction, NAD+ is reduced to NADH+ H+ and this NADH + H+ feeds electrons and protons at the point of origin in the ETC.
- Both e– and protons are transported to FMN which is then reduced to FMNH2.
- FMNH2 transfers only e– to FeS center whereas protons are extruded outside the membrane (intermembrane space), in the process FMNH2 is oxidized back to FMN.
- Electrons flow through FeS centers which alternate between reduced (Fe2+) and oxidized (Fe3+) froms.
- Electrons are finally transferred to ubiquinone, which along with protons obtained by the hydrolysis of water in the matrix site of the membrane is reduced to UQH2.
Complex II: Succinate dehydrogenase complex.
- Complex II is also known as succinate dehydrogenase complex.
- Succinate dehydrogenase complex is located towards the matrix side of the membrane.
- Succinate is oxidized to fumarate as it transfers two e–s and two protons to FAD.
- FAD is reduced to FADH2.
- FAD transfers only electrons through FeS center to quinone.
- Quinone (Q) in presence of protons is reduced to QH2.
- Complex II consists of covalently linked FAD containing flavoprotein and two FeS centers.
Complex III: Cytochrome bc1
- Ubiquinone are hydrophobic, lipid soluble molecules capable of diffusing across the membrane.
- Because of this property, ubiquinones can channel electrons between less soluble electron carriers.
- Electrons are channeled from complex I and complex II to cytochrome bc1.
- The figure shows the stoichiometry for two ubiquinone (UQH2).
- Ubiquinones undergo two rounds of oxidation, one towards the enzyme site on the inner membrane site of the membrane where two electrons are transferred across cyt c1 to cyt c.
- Another oxidation occurs towards the site of membrane containing cyt b where again 2 electrons are passed to cyt bc and cyt bH.
- During these two oxidation reactions, four protons are expelled outside the membrane and 2UQH2 is oxidized to 2UQ.
- One of the UQ diffuse towards the matrix site of the membrane where it receives two electrons flowing through cytochrome b1.
- This UQ along with two protons obtained from the hydrolysis of water in the matrix site of the membrane is reduced to UQH2, thus completing the Q-cycle.
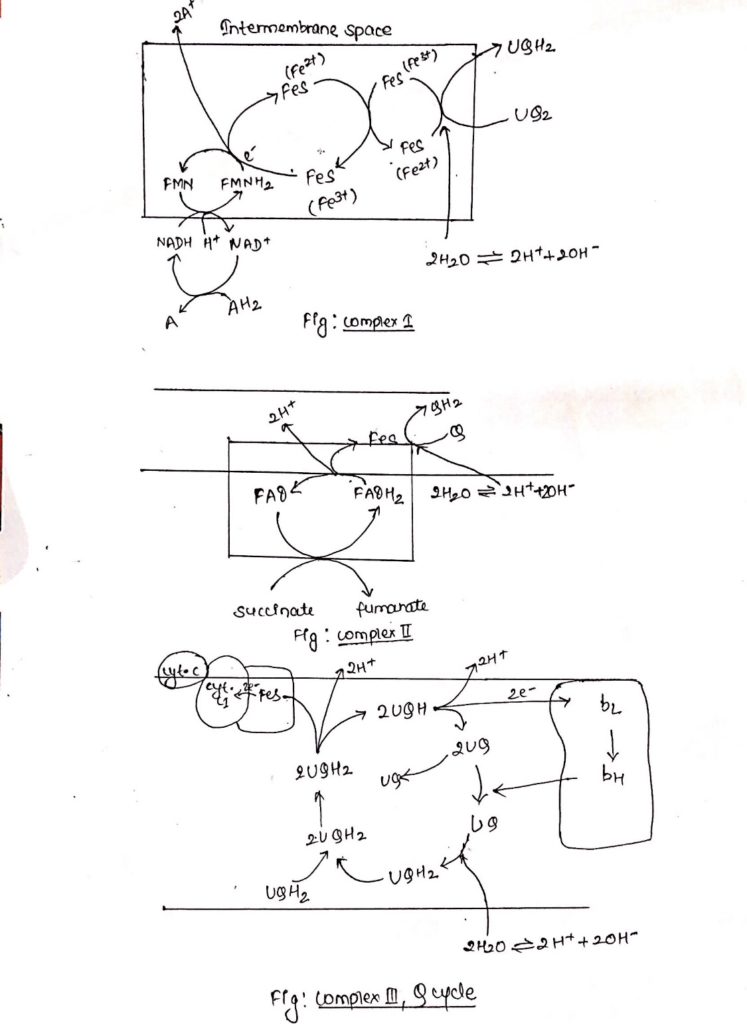
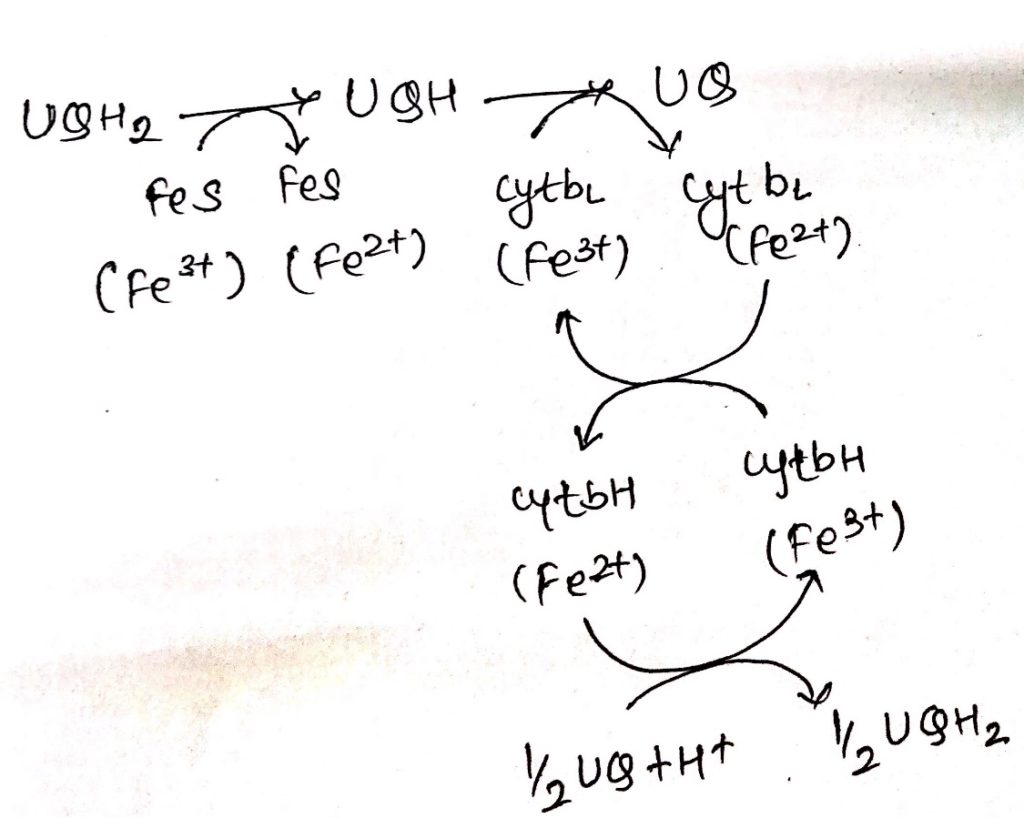
Complex IV: Cytochrome Oxidase
- It is also called as cytochrome oxidase.
- Cytochrome c undergoes oxidation in the side of the membrane facing the intermembrane space and O2 is reduced in the matrix side of the membrane to H2O.
- Complex IV consists of iron containing heme-a and heme-a3.
- Along with iron atoms, cytochrome oxidase also consists of Cu A and Cu B.
- Cu A is closely but not intimately associated with heme ‘a’ and Cu B is intimately associated with heme a3.
- Electrons from cytochrome c flows to Cu A and then to heme ‘a’ and then to heme a3 and then to Cu B and then finally to Oxygen.
- Cytochrome c —> Cu A —–> Heme a—–> heme a3—->Cu B—> O2
- The copper atoms interconvert between cuprous (reduced) and cupric (oxidized).
- Electrons from Cu B and heme a3 is transferred to O2 forming O–-O– bridge.
- Two more electrons are pass through O–-O– resulting in breakage of O–-O– bridge forming O2– and O2-.
- Two protons are supplied from the matrix side forming OH– and OH–.
- Now, addition of two more proton from matrix side resulting in formation of two molecule of water (2H2O).
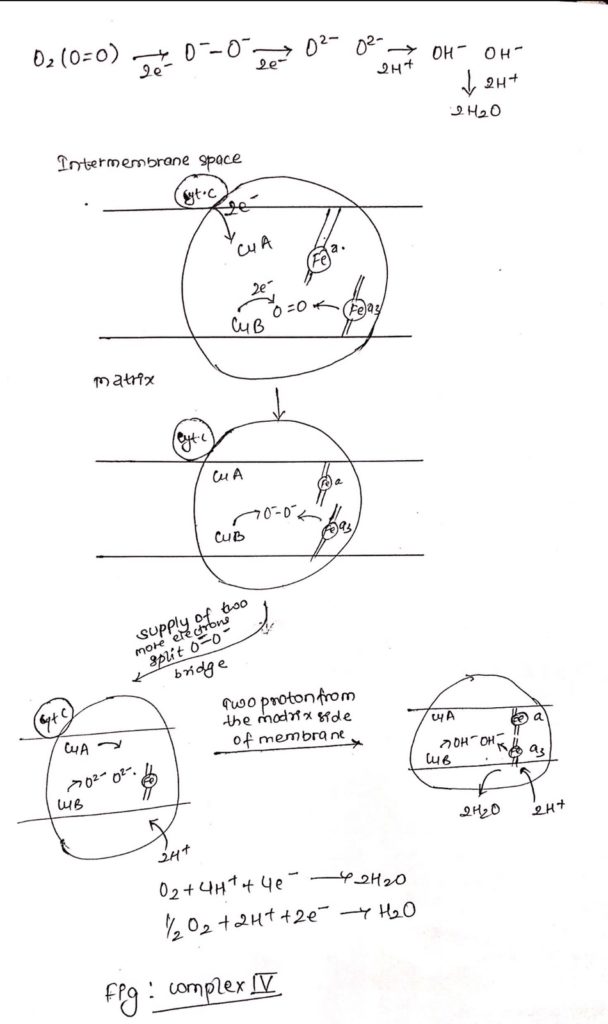
ATP synthesis:
- Chemiosmotic theory given by Peter Mitchell (1961) in the widely accepted mechanism of ATP generation.
- According to this theory electron and proton channel into the membrane from the reducing equivalence flows through a series of electron carriers, electrons flow from NADH through FMN, Q, cytochrome and finally to O2.
- However, proton as they flow through the membrane are extended at different position in the intermembrane space.
- The extension of protons creates a slight positivity/acidity to the outerside of membrane.
- Reduction of quinones and O2 to water requires protons which are provided by the hydrolysis of water in the matrix side of the membrane.
- This results in accumulation of hydroxyl ion in the inner (matrix) side of membrane resulting in slight negativity/alkalinity in the inner side of the membrane.
- This creates a charge difference between outer side of the membrane, and inner side of membrane which energizes the membrane.
- This is electrochemical potential, and this potential along with the pH gradient generates the proton motive force (PMF).
- This proton motive force tends to drive the proteins through ATP synthase in to the inner side of the membrane, the consequence of which is ATP production.
- ATP synthase consists of two components, transmembrane ion conducting subunit called Fo and cytoplasmic multiprotein subunit called F1 which is responsible for ATP production.
- F1 catalyzes the reversible reaction in which ADP is phosphorylated to ATP.
- ADP + Pi <
————->ATP
- ADP + Pi <
- Proton motive force driven H+ through Fo causes the rotation of C-protein of the subunit.
- Rotation of c generates torque.
- This torque is transmitted through
gamma (γ) and epsilon (ε) subunit to β-subunit of F1 resulting in its conformational change.
- This conformational change in
β-subunit allows binding of ADP with inorganic phosphate (Pi).
- Binding of ADP and Pi results in production of ATP and
β-subunit original conformation is regained.
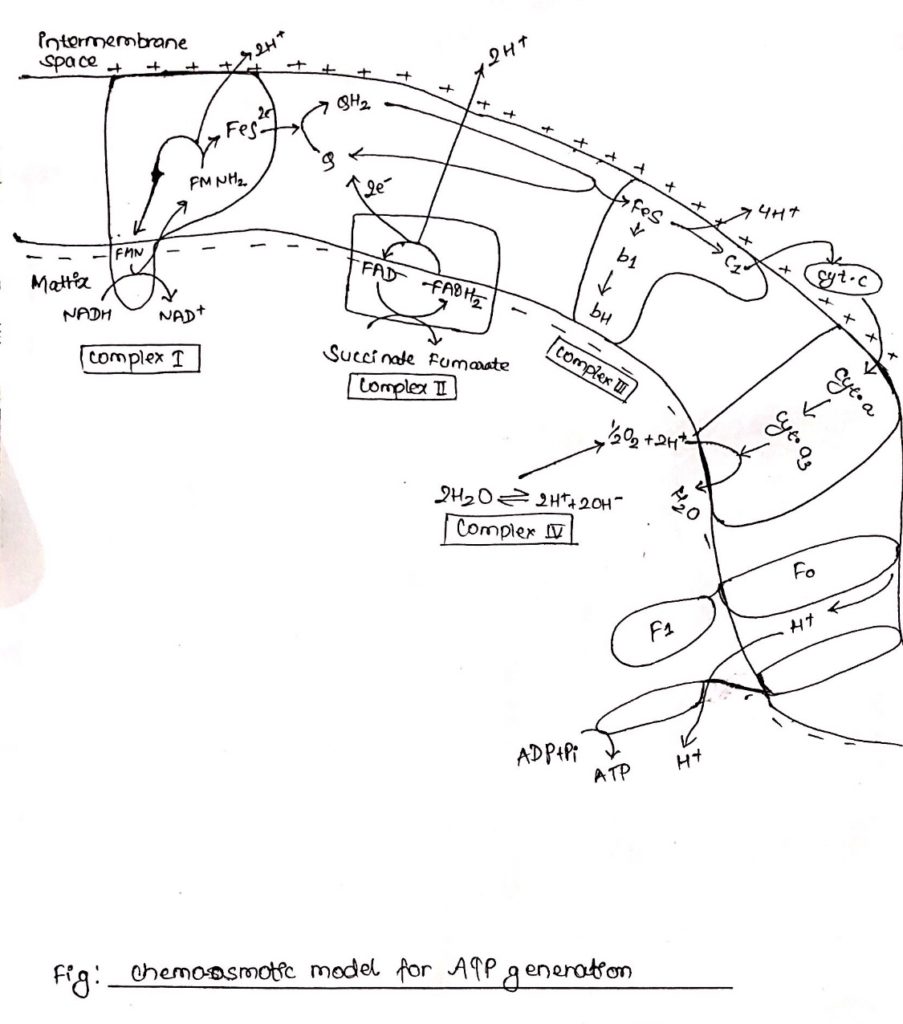